PART II OF VI 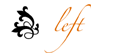 SWEATY SEX by Bethany Lindsay HALF BAKED SCIENCE: A PRIMER ON MEDICINAL CANNABIS by Ryan N. Philippe HEY BABY! I THINK OUR INTERACTION FUNCTIONS WOULD MESH WELL. LET'S DATE by Clive Glover WATCHING THE BIRTH OF THE UNIVERSE: BOOK REVIEW: A SHORT HISTORY OF NEARLY EVERYTHING by David Secko ELSEWHERE AND OVERHEARD by Caitlin Dowling | | | | HALF BAKED SCIENCE: A PRIMER ON MEDICINAL CANNABIS by Ryan N. Philippe images by Jen Philpot Cannabis (marijuana) is among the most widely used of all psychoactive drugs. Despite the fact that its possession and use is illegal in most countries, cannabis is used regularly by as many as 25 million people in North America and Europe and by millions more in other parts of the world. There has been renewed interest in the potential medical uses of cannabis (Cannabis sativa) in recent years, with voters in several areas of Canada strongly supporting such a move. Opinion polls suggest similarly strong popular support for the reintroduction of medical cannabis in the USA, the UK, and many European countries. Expert reviews of medical and scientific evidence on this topic carried out on both sides of the Atlantic in the past few years have encouraged further clinical and scientific research [1-3]. This paper seeks to give a brief review of the scientific facts, ranging from the botany of the cannabis plant and the biochemistry of the receptors it binds, to the potential uses of the plant for medicinal purposes. The Plant Cannabis is one of the very oldest of economic plants, providing fiber, edible seed, and drug resin. The plant likely originated in Central Asia or near the Altai or the Tian Shan mountains. It was first cultivated in China, followed shortly by cultivation in India [4]. Human selection for various uses and natural selection pressures imposed by diverse climates has resulted in a wide variety of growth forms and chemical compositions. Innovative classical breeding techniques have been used to improve drug cannabis, resulting in many cannabinoid-rich cultivars suitable for medical use. The production of cannabinoids is unique to Cannabis, and cultivars with specific chemical profiles are being developed for diverse potential pharmaceutical uses. Cannabis is an annual plant, propagated from seed or vegetative cuttings, and it grows vigorously in open sunny environments as part of its natural life cycle. In light, well-drained soil with ample nutrients and water, the plant will reach up to 5 metres in height in a four- to six-month growth season. Feral Cannabis populations are frequently found in association with human habitation. Agricultural lands, roadsides, exposed riverbanks, meadows, and disturbed lands are ideal habitats for wild and feral Cannabis, as they provide adequate sunlight [4]. Seeds usually germinate in three to seven days. During the first two to three months of growth, juvenile plants respond to increasing day length with more vigorous vegetative growth and new leaf development. Later in the season (after the summer solstice), shorter days (actually, longer nights, if you consider how the photoperiodic effect is monitored) induce flowering and complete the life cycle. Cannabis begins to flower when exposed to short day lengths of 12 to 14 hours or less (long nights of 10 to 12 hours or more) depending on its latitude of origin. However, a single evening of interrupted darkness can disrupt flowering and delay maturation. If an individual plant is not crowded by its neighbours, as in the case of crops intended for seed or drug production, flower-bearing limbs will grow from small buds located at the base of the leaf petioles originating from nodes along the main stalk [4]. Cannabis is normally a dioecious plant, with male and female flowers developing on separate plants. The sexes of Cannabis are anatomically indistinguishable until they begin flowering. However, Mandolino and Ranalli report success using RAPD analysis to identify male-specific DNA markers, to separate out the two sexes before fertilization can occur [5]. The development of male and female plants varies greatly. The male flowers hang in loose clusters along a relatively leafless upright branch. In contrast, the female plant has very crowded clusters of individual flowers at the base of each leaf along a branch. Male Cannabis flowers require air currents to carry pollen grains to the female flowers, which results in fertilization and subsequent formation. The male plants finish shedding pollen and die before the seeds in the female plants ripen 4-8 weeks after being fertilized. The singleseed in each female flower ripens in 3-8 weeks and will either be harvested by humans, eaten by birds or rodents, or it will drop to the ground. A large female plant can produce over one kilogram of seed. This completes the natural four to six month life cycle. If birds or rodents do not consume the seeds, they may germinate the following spring. Cannabis seeds are a balanced source of essential fatty acids, and easily digestible protein. They are usable as human food or animal feed. These essential fatty acids have also been shown to have many important physiological roles and hemp seed oil is a valuable nutraceutical [6]. Much of the Cannabis presently used for medical purposes is grown indoors under artificial lights. Metal halide and sodium vapour light systems are most often set up in attics, bedrooms, or basements. Most modern indoor growers produce vegetatively propagated crops, meaning they are grown from cuttings rather than form seeds. Only female drug Cannabis plants are economically valuable and garden space is often limited. In addition, the legal systems of many nations penalize growers of large quantities of cannabis with harsher penalties. Therefore, under artificial growing conditions, crops are reproduced vegetatively by planting root cuttings of only female plants, or by transplanting and inducing flowering almost immediately. Cuttings taken from one plant are genetically identical copies of a single plant and they should all respond in the same way to environmental inputs. Given that environmental influences are constant, the clones will yield a uniform crop of nearly identical seedless females. Under ideal conditions, yields of dried floral clusters can reach 1,200 grams per square meter per year if three to five crops are grown per year [4]. The psychoactive component of cannabis, D9-THC (D9-tetrahydrocannabinol), is produced in resin glands that adorn the surface of Cannabis leaves. When resin gland development commences, the medically important cannabinoids and the associated aromatic terpenoids begin to appear. Solitary resin glands most often form at the tips of slender stalks which form as extensions of the plant surface. The cluster of one- or two-dozen head cells atop each stalk secrete aromatic terpenoid-containing resin with a very high percentage of cannabinoids (> 80%), which collects under a thin waxy membrane surrounding the secretory head cells [7]. The secreted resin component is, in large part, segregated from the secretory cells. This isolates the resin from the atmosphere as well as membrane-bound enzyme systems within the secretory cells, possibly protecting the terpenoids and cannabinoids from oxidative degradation and enzymatic change. Resin glands containing cannabinoids and terpenoids may have an adaptive significance for the Cannabis plant as defense against environmental challenges, including insect attack or drought [8]. However, Cannabis crops are still subject to infestation by a wide variety of pests, particularly under greenhouse or grow-operation conditions. The intoxicating effects of this Cannabis resin have increased cannabis consumption by humans, as well as encouraged its domestication, thus dramatically widening the distribution of the plant. Cannabinoid Receptor System Because psychotropic cannabinoids have high lipid solubility and low water solubility, they were long thought to owe their pharmacological properties to an ability to perturb the phospholipids constituents of biological membranes. Although this mode of action cannot be excluded altogether, it is now clear that for many of the effects of cannabinoids, the predominant underlying mechanism is far more specific. It is now known that cannabinoids act through receptors: CB1 receptors (cloned in 1990), and CB2 receptors (cloned in 1993) [9]. Both of these receptor types are coupled through G proteins, negatively to adenylate cyclase and positively to mitogen-activated protein kinase. In addition, CB1 receptors are coupled to ion channels through G proteins, negatively to calcium channels and positively to potassium channels. CB1 receptors also mobilize arachidonic acid and close receptor ion channels [10]. 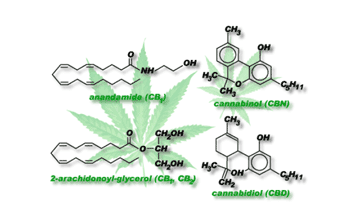 Figure 1. Some examples of endogenous (left) and exogenous (right) cannabinoid receptor ligands. CB1 receptors are found in particularly high concentrations within the central nervous system (CNS). However, they are also present in certain peripheral organs and tissues. These include some neurons and endocrine glands, leukocytes, spleen, heart, and parts of the reproductive, urinary, and gastrointestinal tracts [9]. Some CB1 receptors are located at central and peripheral nerve terminals where they probably modulate neurotransmitter release when activated. Although the concentration of CB1 receptors is considerably less in peripheral tissues than in the central nervous system, this does not imply that peripheral CB1 receptors are not important. Some peripheral tissues may contain high concentrations of CB1 receptors, localized in discrete regions such as nerve terminals that form only a small part of the total tissue mass. Within the CNS, the distribution pattern of CB1 receptors is heterogeneous and can account for several prominent pharmacological properties of CB1 receptor agonists (a chemical that can combine with a receptor on a cell to produce a physiologic reaction typical of a naturally occurring substance). For example, evidence shows that the cerebral cortex, hippocampus, and basal ganglia (areas of the brain involved in learning and thinking) are richly populated with CB1 receptors and that these receptors mediate the well-documented ability of CB1 receptor agonists to impair cognition and memory and to alter motor function [9]. CB1 receptor agonists are also analgesic, and in line with this property, there is evidence for the presence of CB1 receptors in several areas of the CNS that mediate the perception of pain [11]. CB2 receptors are expressed primarily by immune tissues, for example leukocytes, spleen and tonsils [9]. More mRNA for CB2 than CB1 receptors exists in the immune system, and the level of CB2 mRNA in human tonsils matches that of CB1 mRNA in human cerebellum. Levels of CB1 and CB2 mRNA in human immune cells have been shown to vary with cell type [11]. CB2 mRNA has not been detected on neuronal tissue from human or rat brain. In contrast to the CB1 receptor, the physiological roles of CB2 receptors are proving very difficult to determine. The discovery of cannabinoid receptors was followed in 1992 by the demonstration of the existence of endogenous cannabinoid receptor agonists [12]. The most important of these are arachidonylethanolamide (anandamide) and 2-arachidonylglycerol (2-AG), and there is evidence that both of these compounds can serve as neuromodulators or neurotransmitters. This belief comes from demonstrations that they are synthesized by neurons, that they can undergo depolarization-induced release from neurons and that once released they are rapidly removed from the extracellular space [12]. For anandamide, such removal occurs when neurons take the chemical up into the cell from the extracellular space. Once within the cell, anandamide is presumably degraded to smaller signaling compounds that will elicit a physiological effect. Cannabinoid receptors and their endogenous ligands together constitute what is now often referred to as the endogenous cannabinoid system. Current research is exploring the role of the endogenous system in the human body. Hypothesized functions, based on the location where the cannabinoid receptors are found, involve learning, memory, appetite, reproduction, and immunity. Much more work remains to be done in this rapidly expanding field. Medicinal History One of the first crops to be cultivated by mankind, cannabis use is as old as agriculture. First grown for its fibres, the ancient Chinese used it to make rope, cloth and even paper. One of the worlds first pharmacy books, the Pen Tsao, published in China around 2800 BC, recommends hemp as a remedy for just about everything- including, ironically, absentmindedness. The earliest references to its psychoactive properties appear in the Atharva-Veda, a sacred Indian text dating back to 2000 BC [13]. It was probably the Scythians, a barbaric tribe from the Caucasus, who introduced the plant to Europe. The Greek traveler Herodotus (circa 500 BC) wrote of the Scyth warriors purifying themselves in steam baths filled with smoke from burning hemp seeds:They make a booth by fixing in the ground three sticks inclined toward one another, and stretching around them woolen pelts which they arrange so as to fit as close as possible: inside the booth a dish is placed upon the ground into which they put a number of red-hot stones and then add some hemp... immediately it smokes and gives out such a vapour that no Greek steam bath can surpass it... the Scyths howl with pleasure at these baths. Herodotus claims were recently born out by archaeological finds of tripods, braziers and hemp seeds in frozen Scythian tombs in central Asia [13]. The Greeks and Romans cultivated hemp for its fibres, seeds, and medicinal applications, although there are a few references to its use as a social lubricant at banquets to promote hilarity and enjoyment. Throughout the Middle Ages and into the Elizabethan era, hemp was grown in Europe and in Britain, where it fulfilled the massive demand of the British Navy. While the therapeutic properties of cannabis were being all but ignored by the Europeans, in India, entire systems of medicine were being built up around it. The herbs intoxicating effect was very closely tied to its medicinal use. Because of its psychoactive properties it was prized more than ordinary medicines. It was prescribed for a variety of ailments, including headaches, mania, insomnia, rheumatism, menstrual pain and tuberculosis [13]. W.B. OShaughnessy, a surgeon with the British East India Company, and professor at the University of Calcutta, introduced cannabis to modern Western medicine. In 1839, after investigating its use in India and validating many of its applications, he documented its properties as an analgesic in the treatment of rheumatism, and as a remedy for severe convulsions. His contemporary, Jean Joseph Moreau de Tours, a French psychologist, proposed using cannabis as a means to treat mental illness. Interest in cannabis as a medicine spread from Europe to America, and very quickly preparations of cannabis became widely available. The famous physician Sir John Russell Reynolds prescribed it to Queen Victoria for menstrual cramps. Needless to say, its intoxicating nature didnt remain a secret for very long, and many artists and intellectuals of the time readily embraced it [13]. Until the early twentieth century, cannabis was legal and found common use as an everyday medicine. Doubts about the drug began to surface when, at the Second Annual Opiates Conference in 1924, an Egyptian representative complained that workers preferred to lie around smoking hashish than do anything constructive. In 1925, the Dangerous Drugs Act became law, and non-medicinal cannabis was made illegal in Britain. Canada followed suit and banned all forms of cannabis in 1927. Finally, in 1937, with 28 cannabis pharmaceuticals on the American market, the US government effectively criminalized cannabis by passing the Marihuana Tax Stamp Act [13]. Modern Medicinal Applications The recently revived interest in the medical uses of cannabis arose at least partly form its popularity as a recreational drug in the 1960s and 70s. Anecdotal reports from young cancer patients who used cannabis reported that it relieved the nausea and vomiting caused by chemotherapy treatments. Modern chemistry also brought about increased interest in the use of at least one of the constituents of cannabis, whenGoani and Mechoulam isolated and synthesized D9-THC in 1964 [14]. This made the clinical testing of D9-THC during the 1970s and 80s possible, and eventually resulted in the official approval and marketing of the compound as a prescription drug. The chemical synthesis of D9-THC also made possible the synthesis and clinical testing of an entirely new class of pharmaceutical compounds, the synthetic cannabinoids. Dronabinol is another name for the synthetically manufactured (-)-trans-isomer of D9-THC, which is often mentioned in a medical context in scientific literature. Marinol is a trademarked dronabinol preparation dissolved in sesame oil in capsules. It is a registered trademark of Unimed Pharmaceuticals, Inc. It is available in Canada, the USA, and the UK [15]. However, dronabinol has a relatively high incidence of side effects, particularly anxiety and depression. Nabilone is a synthetic derivative of D9-THC with a slightly modified molecular structure. With regard to pharmacological activity, 1 mg of nabilone corresponds to about 10 mg of dronabinol. It is a registered trademark of Eli Lilly & Co., and marketed under the name Cesamet. It is on the market in Canada and the UK, and some other European countries [15]. The Pfizer compound levonantradol was tested in several clinical trials during the early 1980s. It proved to be considerably more potent than morphine as an analgesic, and was effective in blocking nausea and vomiting in patients undergoing cancer chemotherapy. Nevertheless, the psychoactive side effects proved to be unacceptable and the company decided to abandon further research on this project [16]. Other synthetic cannabinoids have been developed with applications relating to research, due to undesirable side effects. Research continues into the development of synthetic cannabinoids that can provide therapeutic benefits while minimizing the medically undesirable psychoactive side effects. Cannabis preparations and synthetic cannabinoids have been employed in the treatment of numerous diseases, with marked differences in the available supporting data. For applications such as nausea and vomiting associated with cancer chemotherapy, anorexia and cachexia in HIV/AIDS, and spasticity in multiple sclerosis and spinal cord injury, there is strong evidence for medical benefits. For indications such as epilepsy, movement disorders, and depression, much less data is available. However, the history of clinical use of cannabis and cannabinoids has demonstrated that the scientific evidence for a specific indication does not implicitly reflect the actual therapeutic potential for a given disease. Clinical studies with single cannabinoids (like dronabinol), or, less often, with whole plant preparations (smoked cannabis or cannabis extract), have often been inspired by positive anecdotal experiences of patients employing crude cannabis (often illegally). The antiemetic properties, appetite enhancing effects, relaxing effects, and therapeutic use in Tourettes syndrome were all discovered (or rediscovered) this way. Movement and spasticity disorders In small clinical trials of THC (dronabinol), nabilone, and smoked cannabis, a beneficial effect on spasticity caused by multiple sclerosis or spinal cord injury has been observed [17]. Among other positively influenced symptoms were pain, paraesthesia (tingling skin), tremor, and ataxia (uncoordinated movement). In folk medicine there are also report of improved bowel and bladder control. Some anecdotal evidence for the benefits of marijuana in spasticity due to central lesions also exists [18]. There are some positive anecdotal reports of therapeutic response to cannabis in Tourettes syndrome, dystonia (frozen muscles), and tardive dyskinesia (jerky, flailing movement). The use of the drug in treating Tourettes syndrome is currently being investigated in clinical studies. Many patients experience a modest improvement; however, some show a considerable response or even complete symptom control. Cannabidiol, another natural cannabinoid found in Cannabis, produced a 20 to 50 percent improvement in five patients with dystonia. In Multiple Sclerosis patients, improvements to ataxia and reduction of tremor have been observed following the administration of THC [19]. Despite occasional positive anecdotal reports, no objective success has been found in Parkinsons or Huntingtons diseases. AIDS In addition to a mountain of anecdotal patientreported evidence, the appetite-enhancing and nauseasuppressing effects of THC have also been observed in clinical trials. In a long-term study of 94 AIDS patients, the appetite-stimulating effect of THC continued for months [18]. Patients tended to retain a stable body weight over the course of seven months, instead of succumbing to the wasting syndrome associated with AIDS. However, the slow onset of action of oral THC, and its high cost and high incidence of side effects, result in many patients preferring herbal cannabis. In addition, some people report better symptom control with cannabis rather than dronabinol, which may be related to the additional cannabinoids, such as cannabidiol, that are found in natural cannabis but not in dronabinol. Unfortunately, the potential respiratory damage resulting from smoke inhalation is at odds with the reduced efficacy of the AIDS patient immune system. Harm reduction research indicates that heating cannabis to temperatures well below combustion (vapourization) yields active cannabinoids with a significant reduction or elimination of the toxic compounds commonly found in cannabis smoke. More research is required but vapourizers appear to substantially reduce what is widely perceived as the leading health risk of cannabis [20]. Cancer Cannabis can act an appetite stimulant, and generally improves the quality of life of terminal cancer patients by helping with pain, nausea, vomiting, and loss of appetite associated with chemotherapy [21]. Treatment of pain and of the side effects associated with chemotherapy is the medical indication for cannabinoids that has been most frequently documented. About 40 studies involving THC (dronabinol, nabilone, other THC analogues, smoked cannabis) have been performed. Most trials were conducted in the 1980s. Oral THC has to be given in high and frequent doses, therefore resultant side effects may occur more frequently than with other drugs. Pain Few clinical studies of cannabinoids effects on pain exist. In two trials, oral THC proved to be effective against cancer pain. However, some patients experienced intolerable side effects. In a single-case double-blind study a patient with recurring pain clearly reduced his need for opiates while receiving THC in comparison to placebo [18]. According to reports from pain therapists, the use of opiates and cannabis concurrently appears to be promising, particularly since cannabis does not cause respiratory depression (as opiates can). Cannabis has been used successfully in modern folk medicine to treat a multitude of painful conditions, such as migraine and other forms of headache, musculoskeletal disorders, arthritis, rheumatism, ulcers, Crohns disease, and menstrual pain. Medical Possibilities Cannabis-based medicines have been used for thousands of years in Asia, and were popular for 100 years in Western medicine after their introduction in the mid-nineteenth century. They fell out of use largely because of the difficulties that physicians encountered in obtaining consistent results from batches of plant material of varying potency. Patients consequently suffered from either treatment with an ineffective dose or the unwanted intoxicating effects of an overdose that could last for many hours. Modern techniques of plant breeding and cultivation have undoubtedly solved the problem of quality control in the use of herbal cannabis as a medicine, but the issue of a narrow therapeutic window between the desired benefits and the usually unwanted psychoactive effects remains a challenge. Many scientists would argue that modern medicines should be single chemical entities wherever possible, rather than complex mixtures of plant constituents. Proponents of herbal cannabis assert that the plant material has advantages over the pure cannabinoid D9-THC. This argument is often confused, however, by the fact that herbal cannabis is usually smoked whereas THC is taken orally. The oral route for THC is notoriously slow and unreliable, whereas smoking is a very efficient way of delivering the drug quickly and in a manner that allows flexible dose control [18]. Smoking, however, carries medical risks. In the short term, the irritant effects of cannabis smoke can lead to bronchitis. In the long term, there is the potential for increased risk of cancers of the lung, airways, and mouth. Although the cancer risk cannot be accurately quantified as yet, it may be one of the most serious potential dangers of cannabis [20]. Better methods for delivering both herbal cannabis and pure THC are urgently needed; perhaps vapourization will prove to be an effective and safe mode of administration. Although there have been suggestions that cannabis has adverse long-term effects on pregnancy, the immune system, fertility, and cognition, the available evidence suggests that these are far less severe than originally thought [16]. The 1990s witnessed major advances in our scientific understanding of how THC and other cannabinoids act on the central nervous system. The discovery that the brain and other organs contain specific protein receptors that recognize the drug and trigger cell responses is analogous to the discovery in the early 1970s of opiate receptors in the brain that bind morphine and other opiates. As with the opiates, the discovery of specific cannabinoid receptors prompted the search for putative naturally occurring chemicals that interact with the receptors. This led to the discovery of anandamide as the first of the naturally occurring endogenous cannabinoids. Although anandamide belongs to an entirely different chemical class than THC, it is bound by the same receptors. These discoveries shed entirely new light on the pharmacology of cannabis. Exploration of synthetic anandamide-like chemicals may be one way to obtain improved cannabinoid-based medicines. The pace of discovery has been so rapid in recent years that it would not be unreasonable to anticipate that more members of the cannabinoid receptor family and additional endogenous cannabinoid substances may yet be discovered in the future. This, in turn, could lead to new therapeutic opportunities, perhaps involving a number of natural cannabinoids that do not bind known cannabinoid receptors but nevertheless have some important pharmacological effects. When cannabis medicines disappeared over half a century ago, this was not perceived in most medical circles as a major restriction of the therapeutic arsenal. However, the situation today is somewhat different. Modern cannabis research and traditional usages along with modern anecdotal reports indicate that cannabis may be the drug of choice for certain patients and conditions. The future will demonstrate whether cannabis in a medicinal context must forever remain a subject for historians. References 1. British Medical Association. Therapeutic uses of cannabis. (Harwood Academic Publishers, Amsterdam, 1997). 2. I nstitute of Medicine. Marijuana and medicine: Assessing the science base. (eds. Joy, J.E., Watson, S.J., and Benson, J.A.), (National Academy Press,Washington, DC, 1999). 3. House of Lords Select Committee on Science and Technology. Cannabis: The scientific and medical evidence. (The Stationary Office, London, 1998). 4. Clarke, R.C., Watson, D.P. Botany of natural Cannabis medicines. in Cannabis and cannabinoids: Pharmacology, toxicology, and therapeutic potential. (eds. Grotenhermen F. and Russo E.), (The Haworth Press, Binghampton, NY, 2002). 5. Mandolino, G., Ranalli, P. Advances in biotechnological approaches for hemp breeding and industry. in Advances in hemp research. (ed. Ranalli, P.), (The Haworth Press, Binghampton, NY, 1999). 6. Pate, D.W. Hemp seed: A valuable food source in Advances in hemp research. (ed. Ranalli P.) (The Haworth Press, Binghampton, NY, 1999). 7. Clarke, R.C. Hashish! (Red Eye Press, Los Angeles, 1998). 8. Pate, D.W. The phytochemistry of Cannabis: Its ecological and evolutionary implications in Advances in hemp research. (ed. Ranalli P.), (The Haworth Press, Binghampton, NY, 1999). 9. Pertwee, R.G. Pharmacology of cannabinoid CB1 and CB2 receptors. Pharmacology and therapeutics, 74(2), 129-180 (1997). 10. Pertwee, R.G. Sites and mechanisms of action. in Cannabis and cannabinoids: Pharmacology, toxicology, and therapeutic potential. (eds. Grotenhermen F. and Russo E.), (The Haworth Press, Binghampton, NY, 2002). 11. Pertwee, R.G. Cannabinoids. in Current understanding, emerging therapies, and novel approaches to drug discovery. (eds. Bountra, C., Mungali, R., Schmidt, W.), (Marcel Dekker Inc., New York, 2003). 12. Di Marzo, V., Melck, D., Bisogno, T., De Petrocellis, L. Endocannabinoids: Endogenous cannabinoid receptor ligands with neuromodulatory action. Trends in neuroscience, 121(8), 1557-1566 (1999) 13. Herer, J., Meyers, J., Cabarga, L. The emperor wears no clothes, (Ah Ha Publishing, Los Angeles, California, 2000). 14. Goani, Y. and Mechoulam, R. Isolation, structure and partial synthesis of an active constituent of hashish. Journal of the American chemical society 86, 1646-1647 (1964). 15. Definitions and Explanations. in Cannabis and cannabinoids: Pharmacology, toxicology, and therapeutic potential. (eds. Grotenhermen F. and Russo E.), (The Haworth Press, Binghampton, NY, 2002). 16. Iversen, L.L. The Science of marijuana. (Oxford University Press, Oxford, UK, 2000). 17. Martyn, C.N., Illis, L.S., Thom, J. Jabilone in the treatment of multiple sclerosis. Lancet 345(8949), 579 (1995). 18. Grotenhermen, F. Review of therapeutic effects. in Cannabis and cannabinoids: Pharmacology, toxicology, and therapeutic potential. (eds. Grotenhermen F. and Russo E.), (The Haworth Press, Binghampton, NY, 2002). 19. Meinck, H.M., Schonle, P.W.A., Conrad, B. Effect of cananbinoids on spasticity and ataxia in multiple sclerosis. Journal of neurobiology 236(2), 120-122 (1989). 20. Bayer, R.E. Therapeutic cannabis as an antiemetic and appetite stimulant in persons with acquired immunodeficiency syndrome (AIDS). In Cannabis therapeutics in HIV/AIDS. (The Haworth Press, Binghampton, NY, 2001). 21. Schnelle, M., Strasser, F. Anorexia and cachexia. in Cannabis and cannabinoids: Pharmacology, toxicology, and therapeutic potential. (eds. Grotenhermen F. and Russo E.), (The Haworth Press, Binghampton, NY, 2002).  Ryan Philippe is a molecular biologist and geneticist, currently working on his Ph.D. in Botany at the University of British Columbia. His actual work involves studying plant-insect interactions, but his interests range from natural products diversity to stellar evolution. He eventually wants to contribute to Mars mission planning (so that he can hitch a ride and finally go home). | | | | For those that prefer a print version, please download our beautiful pdf file. (part i pdf) home (again) about (us) archive (of stuff) submissions (or suggest) notes (on masthead) bioteach (.ubc.ca) 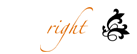 RANK: ANIMAL ILLNESSES by Claire Zulkey CARTOON FEATURE: EINE KLEINE ZELLE. by Corinne Hoesli PARENTS AS A NARCOTIC by Russell Bradbury-Carlin WHITE LADY by PZ Myers A SCIENTIFIC EXPERIMENT by Jaime J. Weinman |