PART I OF VI JULY 11, 2005 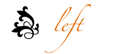 please: pontificate educate illustrate commentate (oh yeah) and/or submit by emailing us at tscq@interchg.ubc.ca | | | | MICROBIAL FUEL CELLS FROM RHODOPHERAX FERRIREDUCENS. By Mario Jardon Novel microbial fuel cells. A recently isolated microorganism has been reported to have a remarkable potential for electricity generation in microbial fuel cells. Rhodoferax ferrireducens, an iron-reducing microorganism, was isolated from subsurface sediments in Oyster Bay, Virginia, USA. Microbial fuel cells composed of this microorganism exceed the performance of previously described microbial fuel cells and even show some clear advantages over existing transition metal-catalyzed fuel cells. Microbial Fuel Cells Before developing the concept of microbial fuel cells, it may be useful to recall the basic concepts of the conversion of chemical energy into electricity. A battery converts chemical energy into electricity through an electro-chemical process. The basic unit of a battery is called a cell. There are three main parts involved in the process: (1) The anode, which is the negative terminal; (2) the cathode, which is the positive terminal; and (3) the electrolyte, which is the ionic conduction medium that allows the ions to travel from anode to cathode. Electrons flow from anode through external load to the cathode (generating electricity). To complete the circuit ions pass through the electrolyte between anode and cathode Fuel cells operate in principle like a battery: they convert fuel to electricity by electrochemical means. However, unlike a battery, a fuel cell does not require recharging since it produces energy as long as fuel is supplied. Hydrogen fuel is fed into the anode of the fuel cell, and oxygen (or air) enters the cell through the cathode. The hydrogen atoms split into protons and electrons, promoted by a catalyst. The protons pass through the electrolyte, whereas the electrons create a separate current that can be used before they return to the cathode to react with the hydrogen and oxygen, forming water. A microbial fuel cell (MFC) is a system that recovers electrons produced during microbial metabolism and channels them for generation of electrical current. MFCs can be divided into three different types (A, B and C). In type A fuel cells, artificial redox mediators are added to the culture in the anodic fuel cell compartment. These mediators penetrate the bacterial cells and transport the electrons to the anode. Type B fuel cells use metal-reducing bacteria, such as members of the families Geobacteraceae or Shewanellaceae, which exhibit special cytochromes bound to their membrane. These are capable of transferring electrons to the electrodes directly. Finally, type C fuel cells oxidize fermentation products (hydrogen, methanol, etc.) on electrocatalytic electrodes, that is, electrodes that have been chemically modified to oxidize such metabolites. 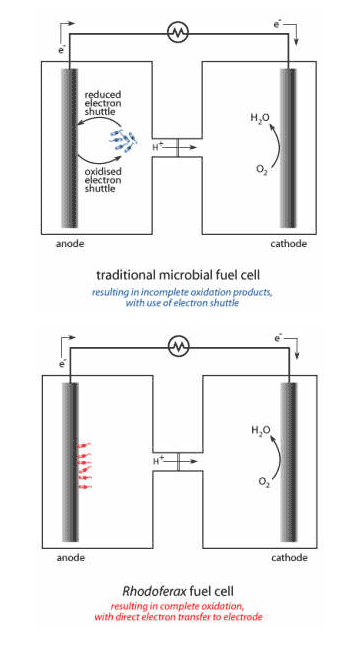 Rhodoferax ferrireducens microbial fuel cell R. ferrireducens reduces Fe(III) during the oxidation of glucose to CO2 and quantitatively transfers electrons to graphite electrodes. It is able to grow at 4 to 30oC (with an optimum at 25oC). Various substrates can support its growth, ranging from organic acids to simple carbohydrates (such as glucose or fructose). Cells in these MFCs are grown under strict anaerobic conditions in a bicarbonate-buffered defined medium, under N2/CO2 (80% and 20%, respectively) at 25oC. For growth on Fe(III), 10 mM Fe(III) chelated with nitrilotriacetic acid (FE(III)-NTA) is provided. The reported MFC is composed of a two-chambered glass vessel connected with a cation-selective membrane with either graphite rods or finely woven graphite felt as electrodes. The liquid volume in each chamber is approximately 210 mL with a headspace of about 160 mL. The anode chamber is continuously flushed with N2/CO2 to maintain anaerobic conditions and maintain the pH balance of the growth medium. The cathode chamber contains 30 mM Tris buffer, and is continuously flushed with sterile water-saturated air. The stoichiometry of glucose oxidation and iron reduction is as follows: C6H12O6 + 6 H2O + 24 Fe(III) -> 6 CO2 + 24 Fe(II) + 24 H+ Potential of R. ferrireducens fuel cells for electricity generation R. ferrireducens has the ability to directly transfer electrons to the surface of electrodes. MFCs using this organism are superior to previously described microbial fuel cells, for various reasons. First of all, this metal-reducing bacterium is able to oxidize glucose at 80% electron efficiency (other organisms, such as Clostridium strains, oxidize glucose at only 0.04% efficiency). In other fuel cells that use immobilized enzymes, glucose is oxidized to gluconic acid, which generates only two electrons, whereas in MFCs using R. ferrireducens, glucose is completely oxidized to CO2. Secondly, these cells do not require the addition of toxic electron shuttling mediator compounds employed in other microbial fuel cells. They also have a remarkable long-term stability, providing steady electron flow over extended periods. Current density of 31 mA/m2 has been produced over a period of more than 600 hours. These MFCs exhibit many of the desirable features of secondary storage batteries, including the ability to be recharged, no severe capacity fading, the ability to accept fast recharge, reasonable cycle life and low capacity loss under open circuit conditions. Finally, they allow the harvest of electricity from many types of organic waste matter or renewable biomass. This fact provides an advantage over other microorganisms in the family Geobacteraceae, which can also transfer electrons directly on electrodes, but which cannot metabolize sugars. Conclusion Even though microbial fuel cells are not yet able to compete with other types of chemically-generated electricity, advancements in this area render this a possibility. Two key challenges in the quest for sustainable societies are energy generation and waste disposal; electrochemical fuel cells, such as the one described in the referenced works can provide a promising solution by linking both tasks. References 1. Chaudhuri S. K., et al., Electricity generation by direct oxidation of glucose in mediatorless microbial fuel cells, Nature Biotechnology, 2003, 21:10, pp 1229-1232. 2. Scholz F. et al, Bacterial batteries, Nature Biotechnology, 2003, 21:10, pp 1151-1152.w  Mario Jardon is a PhD candidate in the Michael Smith Laboratories at the University of British Columbia, who hasn't been in Vancouver long enough to be able to say cool things about himself. | | | | Issue One For those that prefer a print version, please download our beautiful pdf file. home (again) about (us) archive (of stuff) submissions (or suggest) notes (on masthead) bioteach (.ubc.ca) 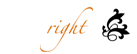 |