THE CELL CYCLE: A UNIVERSAL CELLULAR DIVISION PROGRAM
(August 2003)
In the 17th century, Robert Hooke peered through a microscope at a slice of cork and discovered that it was composed of cells1. Centuries later, Rudolf Virchow set forth the cell theory1, in which he proposed that the cell was the basic unit of all living organisms. We now know that this theory is true; cells make up all living things, whether they are plants, animals, or microorganisms.
This realization produces a reflective concept on the continuity of life. There is only one way to make more cells—by the division of those that already exist. All animals come from animals, all plants from plants, and all cells from cells.
Nature has produced an orderly sequence for the cell to accomplishe this feat. In the simplest sense, a cell duplicates its contents and then divides in two (see Figure 1). The cycle of duplication and division is known as the cell cycle [2].
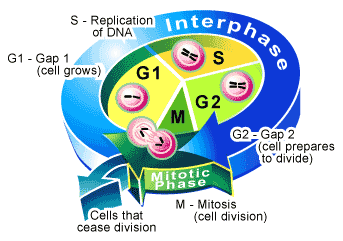
Goal of the Cell Cycle: To produce two genetically identical cells from one precursor cell.
Details of the cell cycle vary from organism to organism and may occur at different times in an organism’s life. Nevertheless, certain aspects are universal2. This is because every cell must accomplish its most fundamental task — copy and pass on its genetic information to the next generation of cells. This requires the replication of the DNA in each chromosome, followed by the acurate separation of the chromosomes into the daughter cells so that each cell receives a copy of the entire genome. Once accomplished, the cell will physically divide to produce two identical daughter cells. Since it is dividing its contents in two, under most circumstances, cells will also double their mass (including duplicating their cellular machinery). This helps cells to avoid the problem of getting smaller each time they divide.
The entire cell cycle will take different amounts of time depending of the organism. For example, cell division in the bacteria (e.g. Escherichia coli) can take as little as 20 minutes [3]. In a single-celled yeast it takes 90-120 minutes [4]. One of the more rapidly dividing mammalian cells has a cycle that takes about 24 hours [5].
The Mammalian Cell Cycle
Using a microscope to watch a mammalian cell reproduce culminates with two visually stunning events: when the nucleus divides (termed mitosis) and when the cell physically splits in two (termed cytokinesis) [2,5].
From start to finish these two events take approximately one hour to complete and together are considered one phase of the mammalian cell cycle called the M phase (M = mitosis). Although M phase is visually dramatic, there is quite a long period between one M phase and another (on average 23 hours), a time that under the microscope appears to be an intermission where the cell merely grows in size.
This period of visual inactivity is called interphase—which on the molecular level is, in fact, quite an active time for the cell. Interphase is divided into the three remaining phases of the cell cycle. During interphase, a cell replicates its nuclear DNA, ensuring that when it does divide at the end of M phase each of the newly created daughter cells will contain a full set of genes. The period in which this is accomplished is called S phase (S = synthesis).
Before DNA synthesis begins and after it is completed, are the two remaining phases of the cell cycle. Since these periods occur between M and S phases they are designated gap phases in which the cell continues to grow. The interval between the completion of M phase and the beginning of S phase is the G1 phase (G = gap), while the G2 phase is the interval between the end of S phase and the beginning of M phase. Gap phases constitute a decision making period for the cell [6] It is within these phases that molecular events within the cell will determine whether to initiate M phase (during G2), S phase (during G1), or to extend the gap for a longer period and allow further cell growth.
Mitosis (Nuclear division)
Separation of the cell’s chromosomes is the key requirement to producing two genetically identical daughter cells, a requirement that is accomplished during M phase of the cell cycle [5].
Chromosomes are normally diffuse strands of DNA that begin to become visible under the light microscope as M phase is about to start. At this stage, the chromosomes, which have been replicated during an earlier S phase, appear as long threads that gradually shrink and thicken so that they are physically easier to separate during mitosis. As the chromosomes condense, a cytoskeletal structure called the mitotic spindle is assembled to carry out their separation (see Figure 2). It is composed of long molecular tubes (called microtubules) that attach to the chromosomes and physically pull them into separate daughter cells.

This occurs in a continuous fashion that has traditionally been divided into five stages (the names of each stage are included below—see Figure 3) [2,5]:
1. The replicated chromosomes condense and the mitotic spindle begins to assemble outside the nucleus (prophase).
2. The membrane surrounding the nucleus (nuclear envelope) breaks down and allows the mitotic spindle to contact the chromosomes (prometaphase).
3. All the chromosomes are gathered at the center of the mitotic spindle (metaphase).
4. The chromosomes are split apart and pulled to opposite sides of the cell (anaphase).
5. The nuclear envelope reassembles around the two new sets of separated chromosomes to form two nuclei (telophase).
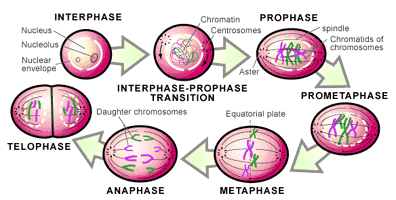
Once the two new nuclei are formed, the chromosomes de-condense and gene transcription can resume (i.e. genes can begin to function). All that remains is for the cell to divide in two.
Cytokinesis (Cell Division)
The second feature of M phase is the time in which the other components of the cell— membranes, cytoskeleton, organelles, and soluble proteins—are distributed to the two daughter cells through a process called cytokinesis. This is the final task that a cell must complete to finish its reproduction (see Figure 4).
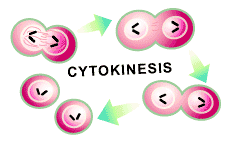
An inward wrinkling of the cell membrane is the first sign of cytokinesis. This is caused by a second cytoskeletal structure called the contractile ring [2], which begins to tighten in that area of the cell. The wrinkle occurs perpendicular to the mitotic spindle that is pulling the chromosomes to opposite sides of the cell and thus ensures cellular division will result in one nucleus in each of the daughter cells.
Once assembled, the contractile ring will tighten further and further until the cell is pinched in two. The force required to do this is provided by the molecular architecture of the contractile ring, which is composed of two types of filaments (called actin and myosin filaments) that slide over each other to create a tightening power [2]. In fact, the contractile ring is capable of exerting a force strong enough to bend a fine glass needle inserted into the cell. This is a transient structure that gradually becomes smaller as cytokinesis proceeds and disassembles completely once the cell is cleaved in two.
The Cell Cycle And Continuation of Life
Once cytokinesis is completed, the cell has successfully gone through one turn of the cell cycle and produced two cells from a single precursor. For bacteria or yeast, which are single-celled organisms, this cell division will produce a new and complete organism. In a multicellular organism (like human beings), a fertilized single-celled egg requires many cell divisions to make a new individual. In either case it is the completion of the cell cycle that produces new organisms, a process that can go on throughout life. In an adult human, for instance, adult nerve and muscle cells do not divide at all. Liver cells divide once a year. More than one division a day occurs in blood cell precursors in the bone marrow and in the cells lining the gut. Our survival requires a production of millions of cells a second. This fact was illustrated by the exposure of organisms to massive doses of x-rays that stop all cell division and cause an individual to die within a few days. In the end, it is the cell cycle that ensures life will always be able to produce more life in an organized fashion.
Texts Consulted and Additional Reading
1. Ford HL, Pardee AB. 1999. Cancer and the Cell Cycle. J Cell Biochem Suppl 32-33: 166-72.
2. Bock et al. 2001. The Cell Cycle and Development. New York: Wiley. 259p.
3. Stein GS, ed. 1999. The Molecular Basis of Cell Cycle and Growth Control. New York: J. Wiley. 389p.
4. Fantes P, Brooks R, eds. 1994. The Cell Cycle: A Practical Approach. Oxford/New York: IRL Press at Oxford University Press. 325p.
5. Cross F, Roberts J, Weintraub H. 1989. Simple and Complex Cell Cycles. Annu Rev Cell Dev Biol 5: 341-395.
6. Jacobs T. 1992. Control of the Cell Cycle. Dev Biol 153(1): 1-15.
7. Dewitte W, Murray AH. 2003. The Plant Cell Cycle. Annu Rev Plant Biol 54: 235-264.
References
1. Serafini A. 1993. The Epic History of Biology. New York: Plenum. 395p.
2. Murray AW, Hunt T, eds. 1993. The Cell Cycle: An Introduction. New York: Oxford University Press. 251p.
3. Vinella D, D’Ari R. 1995. Overview of Controls in the Escherichia Coli Cell Cycle. Bioessays 17(6): 527-36.
4. Forsburg SL, Nurse P. 1991. Cell Cycle Regulation in the Yeasts Saccharomyces Cerevisiae and Schizosaccharomyces-Pombe. Annu Rev Cell Dev Biol 7: 227-256.
5. Norbury C, Nurse P. 1992. Animal Cell Cycles and Their Control. Anuu Rev Biochem 61: 441-470.
6. Gorbsky GJ. 1997. Cell Cycle Checkpoints: Arresting Progress in Mitosis. Bioessays 19(3): 193-7.
(Art by Jane Wang – note that high res versions of image files available here)