THE GENETIC BASIS OF INTELLIGENCE
(August 2004)
Intelligence is a very difficult concept to define. Intellect is described as “the power of the mind to think in a logical manner and acquire knowledge” [1]. Even psychology experts have not agreed upon what this actually means [2]. Intelligence can be divided into various subcategories such as reasoning, problem solving, and memory, and so creating a consistent scale by which one can measure intelligence is quite difficult.
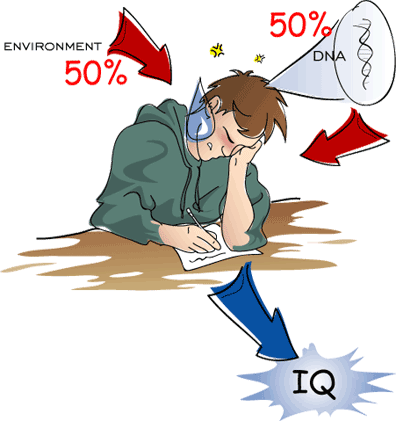
Many researchers working on intelligence use a psychometric definition for intelligence, termed “general mental ability” or the “g factor”. This concept originated from the work of Sir Francis Galton and Charles Spearman [3] of the London School of psychology. Today many psychometricians worldwide accept the theory of general mental ability. According to this theory, on average, those who do well on one mental test also are likely to do well on other mental tests.
In most genetic studies, researchers lump the different subcategories of intelligence into Intelligence Quotient (IQ), a single scale used to quantify intelligence. The concept of IQ was first developed by Alfred Binet, a French psychologist and lawyer, in 1905. In 1914 Stern, a German psychologist, created the measurement scale of IQ by dividing the subject’s mental age by his or her chronological age. Later Terman, a professor at Stanford University, removed the decimal from Stern’s formula by multiplying it by 100. Wechsler developed a similar test for adults called the Wechsler Adult Intelligence Scale [4].
To determine whether scores such as IQs are of genetic or environmental origin, scientific validation is required. Arthur Jensen points to the statistical and biological realities of the g factor. The correlation of g with the overall size of brain, its glucose metabolic rate during problem solving, the complexity and speed of brain waves, as well as estimates of heritability point towards genetic influence [2].
Genetics of Intelligence
The study of intelligence genetics examines how much and by what manner mental abilities are affected by genes. Since many genetic and environmental factors influence intelligence, it is considered a complex trait. However, we do not know much about the quantity and character of genes responsible for mental abilities. We know even less about the factors responsible for expression of these genes.
Intelligence is of primary interest to human genetic research. The first methodical set of experimental observations can be traced back to Galton’s work in 1865, a year before Mendel’s influential article on the laws of heredity [5]. Galton evaluated the transmission of several traits in families using statistical tools. He concluded that many traits including mental ability are genetically transmitted and normally distributed in the general population. He did not analyze the role of the common environment within the families, biasing his conclusions towards the genetic. He did, however, recognize significant general principles such as regression to the mean in children of parents with extreme phenotypic traits.
The first adoptee and twin studies on intelligence were carried out in 1920s. Later animal studies on maze-bright and maze-dull rats investigated individual differences in intelligence. Studies on inbred mice also demonstrated the critical role of genetics in individual differences for aspects of learning. By the 1960s genetic studies on intelligence resulted in declining interest in the environmental origins of intelligence in psychology, enhancing acceptance of a genetic influence on intellect [reviewed in 6]. Then in 1969, in the Harvard Educational Review Jensen suggested that while cultural factors contributed to the 15-point difference in average IQ between black and white Americans, genes could not be ruled out. This declaration made Jensen a target of student protests, acts of vandalism, death threats, and introduced the word “Jensenism” [2]. However, Jensen pointed to an issue that brought strong criticism to genetic research on intelligence, leading to a generation of bigger behavioral genetic studies.
Large sample size studies in monozygotic (MZ) and dizygotic (DZ) twins raised together show an average correlation of .86 for MZ twins, while the correlation for DZ twins is only .60. Twenty-five years later, in The Bell Curve, Herrnstein and Murray [7] claimed that intelligence is strongly inherited with a heritability estimation of .60 + .2 within whites. Based on data from the National Longitudinal Survey of Youth (NLSY), a federal project testing over 10,000 youths in the 1980’s, they declared that social intervention had very little effect on IQ. A large group of scientists and researchers see this claim as racist.
The g factor has a normal distribution in the general population, suggesting g is probably a product of several genes that interact with the environment. Moreover, although g correlates with the parental value, it has a tendency to be closer to the population mean, suggesting a regression to the mean. These observations suggest that some genetic variants that influence g will vary between populations rather than within populations. For instance, certain Asian populations have a frequency of 0.60 in COMT Met158 allele, which predicts lower COMT-enzyme activity and thereby better cognitive performance, while Caucasians have a frequency of 0.42 for the same allele [8].
Studies show a moderate increase in g over time in developed countries, correlating with improvements in nutrition, health and education. Environmental conditions such as socio-economic status have been shown to play a significant role in intelligence. A study by Wahlsten [9] showed that children transferred from a home with low socio-economic status to a home with high socio-economic status improved their test scores as much as 16 points. Moreover, in a study by Plomin et al. there was a .19 correlation for adoptive parents and their adopted children and a .32 correlation for the adopted siblings who had no genetic relatedness, suggesting the shared environment could be responsible for a third of the total variance of g between individuals [6]. It has been reported that heritability for intelligence increases during development, resulting in heritability as high as 80% in adulthood while there is also some evidence that heritability might be lower in adulthood than in childhood [10,11].
Molecular Biology of Intelligence
The correlation between DNA sequence and behavioral differences such as intelligence is considered causal because DNA variations can lead to behavioral differences but behavioral differences do not change DNA sequences [6] . Several methods have been used for DNA research including linkage and association studies.
In contrast to Mendel’s second law of independent assortment, if two genes or a gene and a DNA marker located on the same chromosome are close they can be inherited together within families. This is called linkage. Linkage studies have discovered the location of many single-gene disorders. However, linkage studies are only useful in situations that a single gene has a very large effect: Huntington’s disease, for example.
Cognitive problems including learning disabilities or dementia appear to be complex disorders affected by both multiple genes and environment. Multiple-gene systems result in dimensions (quantitative traits) as opposed to disorders (qualitative dichotomies). Hence, genes in multiple-gene systems are called quantitative trait loci (QTLs). The objective of QTL study is to discover multiple genes with different effect sizes, contributing to the variation of the trait. Improved QTL linkage studies, which use many small families instead of few large ones, can be used to study the extremes of a dimension and are capable of finding genes with more than 10% effect size [6]. Cardon et al.[12] identified the first QTL linkage, a linkage for reading disability.
QTLs can also be identified with a simpler method that can detect QTLs with even much smaller effect sizes on the variance of the trait. This method is called allelic association and detects the correlation between a specific allele and trait in the population.
Benjamin et al. [13] reported one of the first associations for personality: the association between dopamine D4 receptor (DRD4), and novelty seeking. DRD4 gene has two types of alleles, short and long. Novelty seeking scores were higher in subjects with long DRD4 alleles. However, long alleles are also associated with hyperactivity [14]. Association methods have also been used to study diseases such as Alzheimer’s disease [15]. The allele associated with this disease is apolipoprotein E (APOE)-4.
QTLs associated with intelligence are being investigated under the IQ QTL Project. The project focuses on ability rather than disability. The hypothesis of this project is that being very high functioning calls for most of the positive alleles and hardly any of the negative ones. The aim is to use very high functioning individuals to identify QTLs that work all through the total distribution6. Studies of complex traits such as intelligence have 80% power to detect QTLs when they are responsible for 1% heritability. This means that when a single marker is studied, the size of an unselected sample should be about 800. Some of the studies that have used such a large-scale sample size of unselected individuals have reported positive association between normal variation in g and candidate genes including Cathepsin D (CTSD) and cholinergic muscarinic 2 receptor (CHRM2) [16,17] , and the association of catechol-o-methyltransferase gene (COMT) with working memory [18,19]. The studies need to be replicated: Wickelgren et al.[20] reported a positive association between insulin-like growth factor receptor gene with learning and memory but using a larger sample Hill et al.[21] did not find such an association. Moreover, there are many QTLs that are responsible for less than 1% heritability, which means detecting them is difficult.
Functional Genomics
While genomics provides information about what proteins could potentially be in a cell, only functional genomics determines which of those proteins actually exist. Proteins also undergo modifications and interactions that cannot be predicted from genomics alone. Functional genomics operates under three major categories: gene manipulation, gene expression profiling, and proteomics.
In gene manipulation studies the sequence of a gene of interest in an animal model can be deleted, “knocking out” the gene. This method has been used in intelligence related studies. Silva et al. [22], for example, found that knocking out a particular kinase gene interferes with mouse spatial tasks. Another approach involves “knocking down” the genes using antisense DNA, which binds a complimentary RNA and prevents its translation. Using this approach, Guzowski and McGaugh [23] reported the importance of CRE-binding protein (CREB) gene in memory formation. While knocking out or knocking down a gene can affect a behavior this does not mean that the gene causes that behavior: it is possible the gene along with hundreds of others is involved. Conversely, redundancy in living systems can result in little effect when genes are knocked down and out. Moreover, since different knock out strains can show different results, the importance of other genes is evident. Lastly, knocking out or knocking down genes of interest does not exactly parallel natural control behavior.
Gene expression can be determined by the presence of mRNA which could be transcribed into proteins. Microarrays can detect the expression of many genes at the same time. Gene expression depends on the tissue that is sampled, making the approach difficult for human studies because brain tissue is required. Nevertheless, gene expression profiling has been broadly used in mice.
Proteomics is the study the entire protein complement (proteome) of a cell, tissue or organism. The level of mRNA expression is not always a good reflection of protein level, thus proteomics yields a much more accurate snapshot of a cell’s proteome.
Conclusion
There remains a lack of scientific precision for the definition of intelligence, although many scientists use the psychometric definition, g (general cognitive ability). Whether nature or nurture influences intelligence remains a matter of debate between geneticists and environmentalists, and sits at about 50/50. Intelligence appears to be controlled in part by quantitative trait loci (QTLs). Multiple-gene systems like intelligence result in dimensions (quantitative traits) as opposed to disorders (qualitative dichotomies). The objective of QTL study is to discover multiple genes with different sized effects contributing to the variation of the trait. Several methods including linkage and association have been used for discovering the QTLs. Postgenomics holds promise for understanding the genetics of intelligence.
Glossary
psychometricians-mental testing experts
normal distribution– a distribution that can be represented as a symmetrical bell-shaped curve, with the greatest frequency in the center.
adoptee and twin studies– adopted individuals can acquire traits from their adopted family only environmentally, and from their biological parents only genetically, making them ideal for study of environmental/genetic effects. Twins raised apart share genetic material, but have different environments.
effect size-strength of the relationship of two variables, calculated by dividing the difference between two population means by their standard deviation
References
1. Hornby AS. Oxford advanced learner�s dictionary of current English. Oxford, UK. Oxford University Press, 2000.
2. Miele F. What is intelligence? In: intelligence, race, and genetics. Oxford, UK. Westview Press, 2002.
3. Spearman C. (1904). General intelligence objectively determined and measured. American Journal of psychology. 15, 201-292
4. Grasso F. (2002). I.Q.-genetics or environment. Allpsych Journal.
5. Galton F. Hereditary genius: An inquiry into its laws and consequences. London, McMillan/Fontana. 1869/1962.
6. Plomin R and Spinath FM. (2004). Intelligence: genetics, genes, and genomics. Journal of personality and social psychology. 86(1), 112-129.
7. Herrnstein RJ, and Murray C. The Bell Curve: Intelligence and class structure in American life. New York, NY. Free Press, 1994.
8. Winterer G. and Goldman D. (2003). Genetics of human prefrontal function. Brain Research Reviews. 41, 134-163.
9. Wahlsten D. (1995). Increasing the raw intelligence of a nation is constrained by ignorance, not its citizens’ genes. The Alberta Journal of Educational Research. 41 (3), 257-264.
10. McGue M, Bouchard TJ, Jr., Iacono WG, & Lykken DT. Behavioral genetics of cognitive ability: A life-span perspective. In R. Plomin & G. E. McClearn (Eds.), Nature, nurture, and psychology (pp. 59-76). Washington, DC: American Psychological Association, 1993.
11. Finkel D, Pedersen NL, McGue M, & McClearn GE. (1995). Heritability of cognitive abilities in adult twins: Comparison of Minnesota and Swedish data. Behavior Genetics. 25, 321-431.
12. Cardon LR, Smith SD, Fulker DW, Kimberling WJ, Pennington BF, & DeFries JC. (1994). Quantitative trait locus for reading disability on chromosome 6. Science. 266, 276-279.
13. Benjamin J, Li L, Patterson C, Greenburg BD, Murphy DL, & Hamer DH. (1996). Population and familial association between the D4 dopamine receptor gene and measures of novelty seeking. Nature Genetics, 12, 81-84.
14. Thapar A. (2003). Attention deficit hyperactivity disorder: New genetic findings, new directions. In R. Plomin, J. C. DeFries, I. W. Craig, & P. McGuffin (Eds.), Behavioral genetics in the postgenomic era (pp. 445-462). Washington, DC: American Psychological Association.
15. Corder EH, Saunders AM, Strittmatter WJ, Schmechel DE, Gaskell PC, Small GW, et al. (1993, August 13). Gene dose of apolipoprotein E type 4 allele and the risk of Alzheimer’s disease in late onset families. Science. 261, 921-923.
16. Jacobs N, Rijsdijk F, Derom C, Danckaerts M, Thiery E, Derom R, Vlietinck R, van Os J. (2002). Child psychopathology and lower cognitive ability: a general population twin study of the causes of association. Molecular Psychiatry. 7(4):368-74
17. Comings DE, Wu S, Rostamkhani M, McGue M, Iacono WG, MacMurray JP. (2002). Association of the muscarinic cholinergic 2 receptor (CHRM2) gene with major depression in women. American Journal of Medical Genetics. 114(5):527-9.
18. Egan MF, Goldberg TE, Kolachana BS, Callicott JH, Mazzanti CM, Straub RE, Goldman D, Weinberger DR. (2001) Effect of COMT Val108/158 Met genotype on frontal lobe function and risk for schizophrenia. Proceeding of National Academy of Sciences of the United States of America. 98(12):6917-22
19. Malhotra AK, Kestler LJ, Mazzanti C, Bates JA, Goldberg T, Goldman D. (2002). A functional polymorphism in the COMT gene and performance on a test of prefrontal cognition. American Journal of Psychiatry. 159(4):652-4.
20. Wickelgren, I. (1998). Tracking insulin to the mind. Science. 280, 517519.
21. Hill L, Chorney MC, & Plomin R. (2002). A quantitative trait locus (not) associated with cognitive ability in children: a failure to replicate. Psychological Science. 13, 561-562.
22. Silva AJ, PaylorR, WehnerJM, & Tonegawa S. (1992). Impaired spatial learning in alpha-calcium-calmodulin kinase mutant mice. Science. 257, 206-211.
23. Guzowski JF, & McGaugh JL. (1997). Antisense oligodeoxynucleotide-mediated disruption of hippocampal cAMP response element binding protein levels impairs consolidation of memory for water maze training. Proceedings of the National Academy of Sciences of the United States of America. 94, 2693-2698.
(Art by Jen Philpot)