BIODIVERSITY: THE BIG(AND EVEN BIGGER) PICTURE
(August 2003)
“Biodiversity, the planet’s most valuable resource, is on loan to us from our children.”
– Edward O. Wilson
As a recent addition to the English language, with roots in both ecology and evolution, the definition of the word ‘biodiversity’ is still evolving. Zoologists and botanists typically consider biodiversity to refer to the variation and frequency of organisms within a given area, whereas evolutionary biologists may prefer to include in this definition the genes that contribute to the variation within a species as well. In either case, biodiversity can generally be defined as the total composition of evolutionary units in a given environment. The differences in focus are important however, as will be discussed later in an examination of microbial biodiversity.
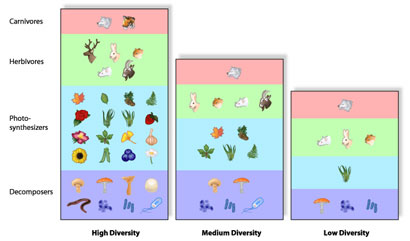
Taxonomists who sought to identify and classify the entire consortium of species in a given environment have traditionally influenced studies of biodiversity. By extensive study of many environments, a list of all of the world’s species could be devised. Studies in this area have consisted of random samplings of a given environment, and have focused primarily on eukaryotic organisms that can be collected and described macroscopically. To date, approximately two million species have been described and named [1]. This gives rise to an estimated 5-10 million species as the total number of eukaryotic species globally. We have a reasonable understanding of most of the birds, mammals, reptiles and amphibians, with respect to their numbers and distribution across the globe. The same is not true of plants, fungi, insects and other invertebrate species – virtually nothing is known of the majority of these, except that they exist.
Lessons on How to Wield Biodiversity
Initially, a catalogue of the world’s species had been conceptualized as a diagnostic tool; tracking changes in the numbers of all the species in a given area could provide insight into the ecological health of that area. Despite their practical application, these studies have declined in popularity in recent years. Although a catalogue of species is necessary in determining how communities change over time, it is generally accepted that, for most well characterized environments, the species that have already been classified can be used as barometers of the overall health of that ecosystem. Thus, in recent years attention has increasingly focused on environmental degradation leading to loss of biodiversity. This shift was born from the realization that the stability of a particular environment’s biodiversity is dependent on far more than preventing any one species from disappearing. For most conservation efforts, results are more easily achieved by protecting an entire habitat.
In the Amazon rainforest for example, rapid deforestation leads to increased runoff and loss of topsoil. Loss of topsoil severely limits the ability of an ecosystem to recover, since topsoil contains the majority of nutrients in the rainforest, and topsoil production typically requires hundreds of years. Since the forests are important for water retention, areas that have been deforested can become deserts, if there is no effort to replant the areas in an attempt to stabilize the soil. Furthermore, the increased organic matter entering waterways can cause problems for the river habitats in the deforested area and anywhere downstream of it.
In addition to these local effects, when rainforests are cleared, they are typically burned. This adds more carbon dioxide to the atmosphere, having the negative consequence of accelerating the greenhouse effect. Between 1980 and 1995, approximately 300 000 km2 of the Amazon basin has been completely deforested [1]. This is equal to the size of all of France. Estimates are that as much as 33 to 50 % of species present in the Amazon basin have already been rendered extinct due to deforestation. This example illustrates why there has been a major shift away from questions of what species are present to studying ways to prevent their extinction. This has led to a much greater public awareness of the importance of biodiversity, for both ecological and economic reasons.
Biodiversity and Ecosystem Vitality
Many of the eukaryotic species that have been studied are well appreciated for the vital roles that they play in maintaining ecosystem health. For example, the large numbers of oysters present completely filters the water of Chesapeake Bay, an area of 7000 square miles, every year. The ecosystem of sand dunes in this area is also completely dependent on the species of grasses that grow there. When the grasses are lost due to human activity, the dunes are destabilized and can disappear completely, and with it, the habitats for numerous small mammals and insects. The inland vegetation may also be damaged by onshore winds that would normally have been blocked by large dunes.
While one species can have an essential role in maintaining an ecosystem’s stability, most ecosystems are much more complicated. More commonly, each species contributes a small amount to the overall health of the ecosystem. This can be conceptualized as most roles within an ecosystem having a certain amount of redundancy. Most ecological roles are fulfilled by several different species – if one of these species is eliminated, the other species will continue to fulfill this niche, albeit to a lesser extent. In this way, biodiversity can be used to represent the health of the complex ecosystem.
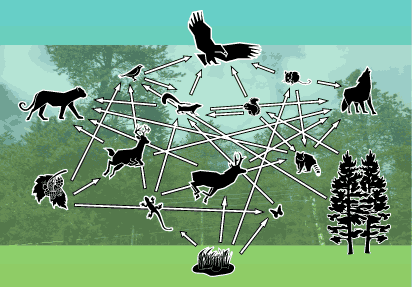
The Star Species in Biodiversity
In these more complex ecosystems, certain species can serve as indicators of the health of that ecosystem. The rationale behind the ‘indicator species’ concept is that certain species are more sensitive to changes in the environment; by carefully monitoring these species’ numbers, one can rapidly detect negative changes within an environment. A species may be used as an indicator, or ‘keystone’ species, given that they fulfill two criteria. Firstly, an indicator species should be extremely sensitive to environmental distress. Indicator species are used to detect ecological imbalance at its earliest stages – choosing an organism that is among the last to suffer in a given ecosystem would be counter productive. Secondly, an indicator species should be relatively abundant in the community being monitored, as this allows for easy sampling. In the Canadian Arctic, these criteria are filled by the polar bear. Recent decreases in the overall mass of polar bears have suggested environmental instability as a result of increased temperatures in the arctic. Elevated temperatures have reduced the number of days that ice cover is present; since polar bears generally hunt for seals at breathing holes in ice floes, the decreased ice cover leads to fewer hunting days per season.
Biodiversity’s Middle Name is ‘Discovery’
While most research has been geared towards conservation attempts, biodiversity plays an essential role in other fields as well. An important aspect of biodiversity has been cataloguing the astronomical number of natural compounds that may have medical or commercial value. Aboriginal cultures have appreciated biodiversity, and the traditional medicines that stem from it, for thousands of years. The value of these traditional remedies has led to the development of an entirely new field of study, ethnobotany; the objective of which is to examine the traditional medicines of native cultures in the hopes of finding new compounds that can be commercially developed.
Several well-known drugs have originated from traditional knowledge. Chewed willow tree bark, used to alleviate aches and tooth pain, led to the discovery and synthesis of acetylsalicylate, one of the world’s most effective pain-relievers and the single best-selling drug in the world [2]. Similarly, a traditional remedy for heart problems led Scottish doctor William Withering to determine that the active ingredient in the remedy was an extract from the leaves of the foxglove plant [3]. Today, the compounds digitoxin and digoxin are still used to control rapid or irregular heartbeats.
Microbial biodiversity, and the attempt to identify new antibiotics has been one of the most important endeavors of the 21st century. The majority of antibiotics in current use are naturally produced by either bacteria or fungi. These organisms produce antibiotic compounds in order to compete with other microorganisms within their environment, particularly soil. The accidental discovery of penicillin in the 1930’s sparked a massive drive to examine thousands of species of bacteria and fungi for new compounds that could also act as antimicrobial agents. This hunt led to the discovery and development of virtually every class of antibiotics that exists to this day by the 1950s. More recent improvement in antimicrobial therapy has come about primarily by chemical modification of compounds that were discovered during this golden age. Despite the success of antibiotic therapy in treating many deadly infections, irresponsible use of antibiotics in both animal husbandry and in human treatment have led to the recent rise in antibiotic resistance to most of these classes of drugs.
Discovery of new bacteria and new antibiotics is still a distinct possibility, despite years of bacterial biodiversity research. This new horizon may be attributed to the discovery that only a very small percentage (0.5 – 2%) of all soil bacteria are culturable [5]. This means that all of the compounds that have ever been recovered from soil microbes have come from approximately 1% of the microbial life in this one environment alone. This observation has led to hope that new life-saving compounds may be discovered from the remaining 99% of soil bacteria. The diversity of life in this environment is truly remarkable, with a single gram of soil containing approximately 108 individual bacteria. However, unlike the study of large eukaryotic organisms, it is much more difficult to learn anything about an organism that cannot be seen nor cultured.
Biodiversity and biotechnology are also seen as intertwined disciplines. The development of one of the most powerful tools in biotechnology, the polymerase chain reaction, or PCR, hinged entirely on a thermostable DNA polymerase isolated from Thermus aquaticus, a thermophilic archaeon first described in the 1960s [4]. This organism was discovered out of a desire to understand the basic biology and biodiversity of Yellowstone National Park. Biotechnology has reciprocated in kind by providing biodiversity research with molecular biology tools as an alternative means of establishing bacterial biodiversity, although this is discussed in further detail later on. Furthermore, this example highlights the importance of conservation efforts for future generations – Yellowstone would almost certainly have been drastically changed in response to human encroachment were it not for the creation of the National Park in 1872.
Defining Bacterial Biodiversity
One of the more difficult problems facing the study of microbial diversity is determining just what a species is. Unlike large sexually reproducing organisms, it is not a trivial decision to determine that a bacterium belongs to a new species. Individual species of animals may differ by as little as 1% in the overall composition of their respective DNA. Among bacteria however, strains that belong to the same species may differ by up to 30%. Consider the “hamburger disease” Escherichia coli O157:H7 and the docile Escherichia coli K12 strain commonly used in microbiological research. The more virulent O157:H7 strain contains approximately 20% more DNA than K12, and yet they are the same species. Since bacteria reproduce asexually, the traditional definition of a species as a single interbreeding population cannot be applied. In fact, bacteria often exchange genetic material in nature among widely divergent taxa, even plants in the case of Agrobacterium.
Thus, for those who study bacterial biodiversity, one of the key questions becomes how do we determine what is present, and once we have determined that, what does each organism do? A great deal of progress has been made regarding which species are present in what quantities. The most powerful tool has been the ability to sequence fragments of DNA from the soil without ever having to culture the actual organism from which they came [1]. PCR has been used to amplify bits of ribosomal DNA that are highly conserved among all bacteria. Differences in these sequences can indicate the type of bacteria that contained it, while the number of copies can be used to approximate how many of that one particular strain were present in the sample.
Determining what each species may be doing is slightly more difficult. In 2000, a group examining ocean-dwelling bacteria found that, contrary to popular belief at the time, rhodopsin proteins are very common in the ocean [6]. Rhodopsins are proteins that capture light energy, which cells later convert into a usable form. Their discovery in marine bacteria suggested that these organisms use light as their major energy source, providing fairly substantial evidence as to what these bacteria do. Furthermore, this group was able to study the newly discovered protein by putting the gene they had isolated encoding for rhodopsin into E. coli. The E. coli bacteria then translated the introduced gene to produce the rhodopsin protein, and in this way, the group was able to avoid the difficulty of not being able to culture the initial marine bacteria. Unfortunately, most bacteria do not present such obvious clues as photosynthetic equipment, and these are still defined as mysterious at best.
Biodiversity is a phenomenon that impacts our lives every day. It plays a role in developing new drugs, discovering new antibiotics, unearthing new biological boundaries, while being a lesson unto itself in its preservation. Apart from these sound reasons, there is also the singular appreciation of how incredibly beautiful an ecosystem is, something to be cherished. Therefore, it is essential that we continue with our efforts to understand different organisms, how they interact, and how we can preserve those interactions for the many years to come.
References
1) Reaka-Kudla, ML, Wilson, DE and EO Wilson, eds. 1997. Biodiversity II: Understanding and protecting our biological resources. Joseph Henry Press, Washington, D.C.
2) Willow bark extract information
3) Mann, R.D., Townsend, H. and J. Townsend. 1986. William Withering and the Foxglove. MTP Press, Boston.
4) Scott, P. 2001. Bioprospecting as a conservation tool: history and background. in Harmon D. (ed). Crossing Boundaries in Park Management: Proceedings of the 11th Conference on Research and Resource Management in Parks and on Public Lands. The George Wright Society, Inc., Michigan.
5) Curtis, TP, WT Sloan, and JW Scannell. 2002. Estimating prokaryotic diversity and its limits. Proceedings of the National Academy of Science. 99: 10494-10499.
6) Beja, O. et. al., 2000. Bacterial rhodopsin: evidence for a new type of phototrophy in the sea. Science. 289:1902-1906.
(Art by Jiang Long – note that high res versions of these image files are available here)