THE COMPASSES OF BIRDS
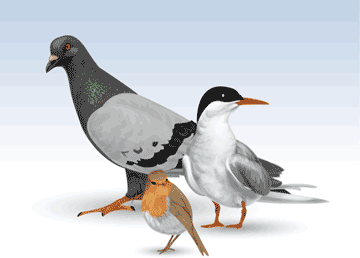
Twice a year, arctic terns make an epic journey. They travel the length of the Earth, from breeding grounds in the high Arctic to their southern habitat in Antarctica and back—a one-way distance of almost 20,000 kilometres[1].
This migratory pattern allows terns to follow their food, and escape extreme winters at both ends of the globe. They also avoid the central latitudes, where the predators are. They have every reason to make the trip, but how do they manage the navigation?
One popular suggestion is that the Earth’s natural magnetic poles help to guide birds in their migrations[2]. How they manage to detect those poles is more difficult to determine.
One theory posits that certain birds’ brains contain magnetite, otherwise known as iron oxide[3]. Magnetite is a naturally occurring magnetic compound that has been found in animals ranging from bacteria to mammals[4]. With physical magnets in their brains, birds would be able to detect the magnetic fields created by the North and South Poles. Magnetite has been found in the upper beaks of several birds, but until recently, there has been little evidence that the substance is connected with navigation[5].
Late last year, a study published in Nature presented evidence that some birds do navigate using magnetite. Homing pigeons earned their name through an ability to “home” in on their lofts, and return to them from hundreds of miles away[6]. It’s not exactly migration, but the distances are comparable.
Homing pigeons had long been thought to navigate either by scent or magnetic attraction. In the latest study, researchers found that they could train pigeons to recognize when an anomalous magnetic field was present—the birds would hop to one end of the cage when the field was normal and to the other when the field became abnormal[6]. In other words, they were detecting differences in the magnetic field.
The pigeons were left to try the same trick with magnets attached to their upper beaks, and could no longer perform the stunt[5]. They also failed when their olfactory cavity (the proposed location of the magnetite) was frozen. Everything that occurred in this study had been seen before in rainbow trout, a species that manages migrations of incredible distances using magnetite found in its olfactory cavity[7].
But if pigeons use magnetite to navigate, they may be an aberration among birds. Most other evidence seems to point to a different technique for sensing poles.
This alternative technique is called a radical-pair mechanism. It is a complex idea that involves electrons spinning around a nucleus in an atom. Electrons come in pairs, and each pair is given an orbit around the nucleus. The two electrons spin in opposite directions—one up and one down, or one north and one south. Each of the electrons is essentially is own magnet, but they neutralize each other’s effects[8].
But free radicals, formed when larger molecules split, do not have a complete set of electrons. One of the electrons is unpaired. These molecules are unstable, and they react quickly with other molecules to form compounds[8].
When two free radicals come together to build a new molecule, they’re constrained by the directions that their unpaired electrons spin. If one has an unpaired “up” electron and the other a “down”, there’s no problem. The electrons are balanced and the radicals will fuse seamlessly[8].
If both unpaired electrons are “up”, on the other hand, the result is much like trying to touch the “north” ends of two magnets together. One of the electrons needs to change its direction—not a simple matter, but not impossible, either[8].
Merging the two radicals becomes more difficult when an external magnetic field is applied[8]. In the radical-pair hypothesis of bird navigation, birds detect the magnetic pull of the North and South Poles by sensing the relative effort of creating stable molecules from two free radicals.

Scientists are able to differentiate between magnetite and radical-pair mediated magnetism in animals because radical-pairs are sensitive to very weak magnetic fields. Weaker fields are dampened by the cellular environment that surrounds magnetite particles, so the fields cannot be detected[9].
In a recent experiment, European robins were conditioned by simulated light conditions to believe that it was time for spring migration[9]. The birds were restless and eager to fly in a northerly direction.
However, when the birds were exposed to weak magnetic fields, they became disoriented and began to fly in all directions. They only faced north when the simulated field was parallel to the Earth’s own magnetic field[9].
The simulated fields were more than 600 times too weak to be detected by magnetite particles, suggesting that radical pairs were involved instead[9]. The scientists did caution that both the radical-pair mechanism and magnetite could be involved in European robin migration.
For a radical-pair mechanism to work, of course, birds need to have radical pairs in their bodies. This may be accomplished by the changing daylight hours during migratory seasons. Both visible and ultraviolet light can produce free radicals.
The proper light conditions are essential for bird navigation. Birds can’t orient themselves correctly in yellow light[10], or in red light without lengthy pre-exposure[11]. Exposure to blue or green light is necessary for birds to begin migrating in the correct direction[10].
The complete theory is that light in the blue-green range excites molecules to create free radicals, which in turn begins the process of magnetic field detection. In plants, cryptochromes are the receptors for blue-green light, and many of the molecules within them will split into radicals when struck by light[12].
Birds are thought to use cryptochromes in some part of their heads to sense magnetic fields. Birds will often turn their heads from side to side a few times before flying off under normal conditions. When an abnormal magnetic field is present, they twist their necks as many as triple the number of times before take-off[13].
Several cryptochromes have been found in the retinas of birds, and a few of these are exclusive to migratory species[14,15]. Garden warblers, a night-migrating species, have particularly high concentrations of a specific cryptochrome located in specific nerve cells that are very active at night, when the birds need to orient themselves[15]. Another cryptochrome found in the same species concentrates in an area that is connected to neurons that are sensitive to magnetism[15].
Birds appear to be using a radical-pair mechanism, mediated by light sensing cryptochromes in their eyes, to sense magnetic fields and keep themselves pointed in the right direction during long migrations. Experiments have also shown that birds recalibrate their compasses once a day to insure that they are flying in the correct direction[16].
The mystery of bird migration probably doesn’t have a simple solution. For migratory birds, travels across land and water seem to be complicated processes involving spectrums of light, electron excitation, and constant recalibrations.
References
1.Salmonsen, F. 1967. Migratory movements of the arctic tern (Sterna paradisaea) in the southern ocean. Bio. Medd. Dan. Vid. Selsk 24, no. 1.
2.Wiltschko, W., and R. Wiltschko. 1972. Magnetic compass of European robins. Science 176: 62-64.
3.Kirschvink, J., and J. Gould. 1981. Biogenic magnetite as a basis for magnetic field detection in animals. Biosys. 13: 181-201.
4.Maugh, T. H. 1982. Magnetic navigation an attractive possibility. Science 215: 1492-1493.
5.Beasons, R. C., and J. E. Nichols. 1984. Magnetic orientation and magnetically sensitive material in a transequatorial migratory bird. Nature 309: 151-153.
6.Mora, C. V., Davison, M., Wild, J. M., and M. M. Walker. 2004. Magnetoreception and its trigeminal mediation in the homing pigeon. Nature 432: 508-511.
7.Diebel, C. E., Proksch, R., Green, C. R., Neilson, P., and M. M. Walker. 2000. Magnetite defines a vertebrate magnetoreceptor. Nature 406: 299-302.
8.Scaiano, J. C. Control of chemical reactions with magnetic fields. Interscientia. Available online. Accessed Mar. 22, 2005.
9.Ritz, T., Thalau, P., Phillips, J. B., Wiltschko, R., and W. Wiltschko. 2004. Resonance effects indicate a radical-pair mechanism for avian magnetic compass. Nature 429: 177-180.
10.Wiltschko, W., Gesson, M., Stapput, K., and R. Wiltschko. 2004. Light-dependant magnetoreception in birds: interaction of at least two different receptors. Naturwissenschaften 91: 130-134.
11.Wiltschko, W., Moller, A., Gesson, M., Noll, C., and R. Wiltschko. 2004. Light-dependant magnetoreception in birds: analysis of the behavior under red light after pre-exposure to red light. J. Exp. Biol. 207: 1193-1202.
12.Giovani, B., Byrdin, M., Ahmad, M., and K. Brettel. 2003. Light-induced electron transfer in cryptochrome blue-light photoreceptor. Nat. Struct. Biol. 10: 489-490.
13.Mouritsen, H., Feenders, G., Liedvogel, M., and W. Kropp. 2004. Migratory birds use head scans to detect the direction of the earth’s magnetic field. Cur. Biol. 9: 1946-1949.
14.Moller, A., Sagasser, S., Wiltschko, W., and B. Schierwater. 2004. Naturwissenschaften 91: 585-588.
15.Mouritsen, H., Janssen-Bienhold, U., Liedvogel, M., Feenders, G., Stalleicken, J., Dirks, P., and R. Weiler. 2004. Cryptochromes and neuronal-activity markers colocalize in the retina of migratory birds during magnetic orientation. Proc. Nat. Acad. Sci. 101: 14294-14299.
16.Cochran, W. W., Mouritsen, H., and M. Wikelski. 2004. Migrating songbirds recalibrate their magnetic compasses daily from twilight cues. Science 304: 404-408.
(Images by Jane Philpot)