CELL SURFACE RECEPTORS: A BIOLOGICAL CONDUIT FOR INFORMATION TRANSFER
The behavior of a cell often relies on the chemical signals it is exposed to in its environment. In general, two types of chemical signals can be found floating through the cellular environment: water-soluble (hydrophilic) molecules or fat-soluble (hydrophobic) molecules. Both are important components of cellular communication and physically interact with cellular structures to facilitate this function. In virtually every case, the cellular structures that these chemical signals interact with are receptor proteins.
Receptors and the Surface of a Cell
A cell is surrounded by a membrane, which forms a barrier between the inside and outside of the cell. This structure consists of a double layer of phospholipid molecules interspersed with cholesterol and proteins (many of which are receptors for chemical signals) that float in or are attached to the membrane. Its fat-rich composition provides it with the characteristic of being selectively permeable, allowing the flow of fat-soluble substances across it, while excluding many water-soluble ones. Fat-soluble signals, such as hormones and some vitamins, are thought to simply diffuse across the membrane. Their receptor proteins are usually found within the cell. In contrast, the vast majority of chemical signals are water-soluble and thus unable cross the fatty lipid layer of the cell membrane. Their receptor proteins must therefore span the membrane, detect their presence on the outside of the cell, and transmit this information into the interior of the cell. These proteins are called cell-surface receptors and can be thought of as a conduit for the transfer of information from the outside of the cell to the inside of the cell (see Figure 1).
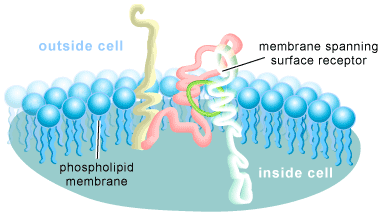
Interactions Between Signals and Receptors are Highly Specific
In the media surrounding a cell, there are hundreds of chemical compounds. They are floating by, bouncing around, and rubbing against the cellular membrane. During this process, many of these compounds will make contact with cell-surface receptors, only to be sprung back into the media with no effect on the cell. The insensitivity that a cell-surface receptor shows for the mix of chemical compounds surrounding it is a result of the high specificity it has for its chemical signal. This specificity is directly related to the molecular contours of the receptor and the signal, so that they fit each other like a lock and key, becoming chemically bonded in the process. Once bonded, the receptor will transmit the presence of the signal into the cell
Classification of Cell-Surface Receptors
Although cell-surface receptors differ in the way they transmit information into the interior of the cell, in mammalian cells most can be generalized into three distinct and large families based on the mechanism they use to accomplish this transmission:
1. Ion-Channel-Linked Receptors: Converting Chemical Signals to Electrical ones
These are fast acting receptors, exemplified by the nervous system that allow sub-millisecond transmission times across synapses. Chemical signals in the form of neurotransmitters are transduced by ion-channel-linked receptors directly into an electrical signal in the form of a voltage difference across the plasma membrane. This occurs when a neurotransmitter binds to this type of receptor, altering its conformation to open or close a channel (often through or near the receptor) to the flow of Na2+, K+, Ca2+, or Cl- ions across the membrane. Driven by their electrochemical gradient (i.e. one side of the membrane has numerous ions, while the other side has few) the ions rush into or out of the cell, creating a change in the membrane potential due to the positive or negative nature of the ions. This flow of ions through the channel can trigger a nerve impulse, or alternatively stop one from occurring. (see figure 2)
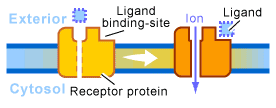
2. G-protein-Linked Receptors: Clicking On Internal Switches
With hundreds of members already identified, this is the largest family of cell-surface receptors. Equally large is the diversity of chemical signals that act through G-protein-linked receptors. Some neurotransmitters that bind to ion-channel-linked receptors also bind to G-protein-linked receptors (although in a much slower fashion). Despite the diversity of signal molecules that bind them, all G-protein-linked receptors studied to date consist of a single polypeptide chain that threads back and forth across the lipid bilayer seven times. The binding of a signal to this receptor results in the switching “on” of a G-protein on the internal face of the membrane. Once activated, this G-protein will initiate a process that will alter cellular behavior. Rhodopsin, the light-activated photoreceptor in the vertebrate eye, as well as the olfactory receptors in the vertebrate nose, are members of this family. It is an ancient protein in terms of evolution, and has been found in organisms ranging from humans to yeast. There are structurally similar proteins found even in bacteria, such as bacteriorhodopsin. (see Figure 3)
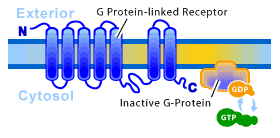
3. Enzyme-Linked Receptors: Directly Activating Biochemical Activity
The study of various growth factors (extracellular signal proteins that regulate growth) shone a light on enzyme-linked receptors when it was discovered that they utilized these receptors. The responses to growth factors are typically much slower (on the order of hours) than responses connected to the other two families of cell-surface receptors. Although, recent studies have begun to connect enzyme-linked receptors to direct effects on the cytoskeleton, envolving cell movement and change of shape. Enzyme-linked receptors only span the membrane once (as opposed to seven times for G-protein-linked receptors). The internal side of the receptor acts as an enzyme, which is activated when the appropriate ligand binds to the external portion of the receptor. The largest class of receptors with this family act as tyrosine protein kinases, which phosphorylate tyrosine side chain residues on selected intracellular proteins. Such receptors are called receptor tyrosine kinases. Their function is essentially quite simple. The binding of a signal to the outer-membrane portion causes the internal kinase to switch on and begin phosphorylating cellular proteins. These phosphorylated proteins will go on to affect responses to the original signal. (see Figure 4)
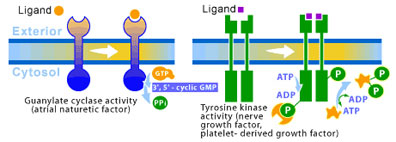
Disease and Cell surface Receptors
Several human diseases have been linked to alterations in cell-surface receptors. These surface receptors activate intracellular signaling cascades. These signaling cascades regulate numerous cellular functions from cell division to cell growth and development. Defects in these proteins (usually stemming from defects in the genes that encode them) can result in disease.
The case of G-protein coupled receptors is an illustrative example. Cholera and pertussis toxins were the first to show how a GPCR could be involved in disease. In this case, the GPCR in question is the bacterial toxin that covalently modifies G protein subunits. After this discovery, many mutations in GPCRs were linked to different disorders. Defective signal of a GPCR can result from many different changes in the normal function of the receptor, such as changes in expression levels, post-translational modification, or mutations. These mutations can occur as somatic events or they can be inherited. How these mutations affect a person will depend on the origin of the mutation. In the case of a germline mutation, generalized manifestation occurs. Somatic mutations result in more focused manifestation. Some germline mutations are incompatible with normal embryonic development.
Mutations in GPCR are known to cause several disorders associated with vision. There are four visual pigments in the human eye: rhodopsin (the rod photoreceptor pigment) and the blue, green, and red cone opsins.
Reference
Spiegel AM. Defects in G-Protein-Coupled Signal Transduction in Human Disease. Annu Rev Physiol 1996; 58:143-70.
(Art by Jane Wang – note that high res versions of image files available here)