PROTEIN PHOSPHORYLATION: A GLOBAL REGULATOR OF CELLULAR ACTIVITY
(August 2003)
As early as the 19th century it was known that phosphates could be bound to proteins. Most examples of these ‘phosphoproteins’ were found in milk (caseins) and egg yolk (phosvitin) and were simply considered a biological method of providing phosphorus as a nutrient. Therefore, the existence of phosphoproteins was considered a consequence of metabolic reactions, and nothing more, for almost a century after their discovery [1].
In the 1950’s this all began to change as phosphoproteins began to emerge as key regulators of cellular life. An initiating factor of this emergence occurred in 1954, when an enzyme activity was observed that transferred a phosphate onto another protein [2] -a biological reaction called phosphorylation. The protein responsible was a liver enzyme that catalyzed the phosphorylation of casein and became known as a protein kinase (see Figure 1), the first of its kind to be discovered. A year later, the role of phosphorylation became more interesting as Fischer and Krebs [3], and Wosilait and Sutherland4, showed that an enzyme involved in glycogen metabolism was regulated by the addition or removal of a phosphate, suggesting that reversible phosphorylation could control enzyme activity. This idea was later proven to be true and has now seeped into virtually every aspect of cell biology.
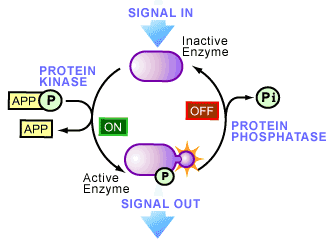
Today, it is thought that one third of the proteins present in a typical mammalian cell are covalently bound to phosphate (i.e. they are phosphorylated at one time or another). The study of cell biology is now littered with examples of regulation by phosphorylation: increasing or decreasing the biological activity of an enzyme, helping move proteins between subcellular compartments, allowing interactions between proteins to occur, as well as labeling proteins for degradation. The variety is immense and now many human diseases have been recognized to be associated with the abnormal phosphorylation of cellular proteins.
These developments have brought the study of phosphorylation into the limelight of medical research, a fact that was recognized in 1992 when Fischer and Krebs received the Nobel Prize in medicine for their pioneering efforts. For those interested, the lectures given by Fischer and Krebs upon receiving the Noble Prize are an excellent overview of the early days of this field [5].
How can phosphorylation control enzyme activity?
Phosphorylation refers to the addition of a phosphate to one of the amino acid side chains of a protein. Remember that proteins are composed of amino acids bound together and that each amino acid contains a particular side chain, which distinguishes it from other amino acids. Phosphates are negatively charged (with each phosphate group carrying two negative charges) so that their addition to a protein will change the characteristics of the protein. This change is often a conformational one, causing the protein to change how it is structured (see Figure 2).
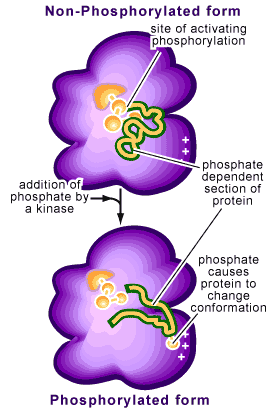
This reaction is reversible by a process called dephosphorylation. The protein switches back to its original conformation when the phosphorus is removed (see Figure 2). If these two conformations provide the protein with different activities (i.e. being enzymatically active in one conformation but not the other), phosphorylation of the protein will act as a molecular switch, turning the activity on or off.
The transfer of phosphates onto proteins is catalyzed by a variety of enzymes in the cell. Although the variety is large, all of these enzymes share certain characteristics and fall into one class of proteins, called protein kinases [6]. Their similarities stem from the group’s ability to take a phosphate off the chemical energy-carrying molecule ATP and place it onto an amino acid side chain of a protein (see Figure 3). The hydroxyl groups (-OH) of serine, threonine, tyrosine or histidine amino acid side chains are the most common target. A second class of enzymes is responsible for the reverse reaction, in which phosphates are removed from a protein. These are termed protein phosphatases.
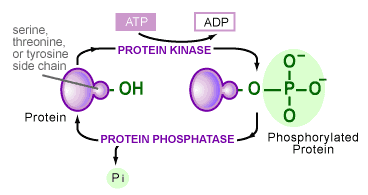
The use of the phosphorylation/dephosphorylation of a protein as a control mechanism has many advantages:
- It is rapid, taking as little as a few seconds.
- It does not require new proteins to be made or degraded.
- It is easily reversible.
The extensive use of this control mechanism is apparent by the large number of known kinases and phosphatases [6]. Even in a simple organism like yeast, approximately 3 percent of its proteins are kinases or phosphatases. Some of these enzymes are extremely specific, potentially phosphorylating or dephosphorylating only a few target proteins, while others are able to act broadly on many proteins. The examples of known targets of phosphorylation include most protein components of the cell, including enzymes, structural proteins, cell receptors, ion channels and signaling molecules. If a protein is controlled by its phosphorylation state, its activity at any one time will be directly dependent on the activity of the kinases and phosphatases that act on it. It is quite common for a phosphate group to be added or removed from a protein continually, a cycle that allows a protein to switch rapidly from one state to another.
External Signals can activate protein kinases and phosphatases
The reception of a signal on the surface of a cell often results in the activation of kinases and phosphatases [6]. Once activated, cellular phosphorylation patterns will begin to change, with various proteins being phosphorylated or dephosphorylated. The final result will be various changes in cellular behavior.
Many of the proteins that are phosphorylated upon reception of a signal are protein kinases as well. This organization of kinases produces a phosphorylation cascade [6] (see Figure 4), in which one protein kinase is activated by phosphorylation upon reception of a signal, this kinase then phosphorylates the next kinase in the cascade, and so on until the signal is transmitted through the cell. In such a system, the kinase cascade can start with the receptor itself (which is often a kinase) or a free-floating cytoplasmic kinase. Upon reception of a signal, these phosphorylation cascades continue to function until protein phosphatases are activated and shut off their transmission.
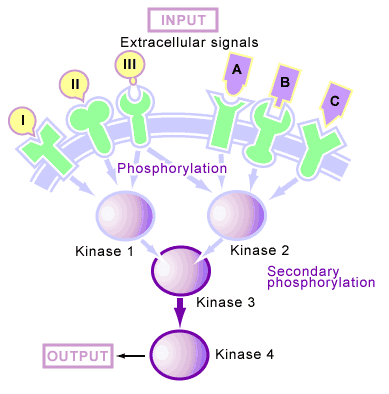
In animal cells, these cascades are mediated by two types of kinases: serine/threonine kinases [6] (which phosphorylate serine and threonine amino acid side chains) and tyrosine kinases [6] (which phosphorylate tyrosine amino acid side chains).
Phosphorylation in response to a signal produces a second outcome apart from the activation of kinases and phosphatases, which involves the production of binding sites for proteins to interact [6]. This process is different from the activation of a protein by phosphorylation (in which the addition of a phosphate causes differences in enzyme activity), since it does not necessarily change the inherent activity of the molecule that has been phosphorylated. Instead, it creates a phosphorylated amino acid on the molecule that another protein can bind to (see Figure 4). Upon reception of a signal, some membrane-bound receptors will become tyrosine phosphorylated. Free-floating proteins then bind to these phosphotyrosine sites and are thus concentrated near the receptor. This concentration often leads to the activation of additional proteins by bringing together molecules that normally would not be in close proximity.
Through the use of phosphorylation cycles and cascades, the cell is able to regulate a diverse set of processes, including cellular movement, reproduction and metabolism. It is the simplicity, reversibility and flexibility of phosphorylation that explains why it has been adopted as the most general control mechanism of the cell.
Addition Reading And Texts Consulted
1. Pawson T. 1994. Introduction: Protein Kinases. FASEB J. 8:1112-1113.
2. Hardie DG, ed. 1999. Protein Phosphorylation: A Practical Approach. Oxford/New York: Oxford University Press. 431p.
3. Hardie DG, Hanks S, eds. 1995. The Protein Kinase Factsbook. London/San Diego: Academic Press. Vol. 1-2.
References
1. Marks F, ed. 1996. Protein Phosphorylation. Weinheim: VCH.
2. Burnett G, Kennedy EP. 1954. J. Biol. Chem. 211: 969-980.
3. Fischer EH, Krebs EG. 1955. J. Biol. Chem. 216: 121-132.
4. Sutherland EW, Wosilait WD. 1955. Nature 175: 169-170.
5. Nobel e Museum: Nobel Prize in Physiology or Medicine (1992):
6. Sefton BM, Hunter T. 1998. Protein Phosphorylation. San Diego: Academic Press.
(Art by Jane Wang – note that high res versions of image files available here)